1999 Leonid Meteor Storm - How the Prediction Fared
Tom Van Flandern -- MRB 99/12/15
Abstract. Our 1999 September 15 issue carried a prediction for the possible 1999 November Leonid meteor storm. We briefly review what the prediction was, how it differed from other predictions, what it was based on, how it fared compared to other predictions, and the reaction of the astronomical community. See summary in Table 1 near end of report.
In the September MRB, Esko Lyytinen of Finland threw his hat into the ring of predictions of the never-before-successfully-predicted Leonid meteor storm phenomenon. In so doing, he joined about a dozen professional astronomers, each using different approaches and each arriving at somewhat different results. Lyytinen and many of the others were motivated by the 16-hour time error and completely wrong rate estimates for the 1998 Leonids that resulted in some astronomers traveling to the wrong side of this planet in unsuccessful attempts to view a possible storm (A meteor storm is defined as a maximum sustained rate of more than 1000 meteors per hour.) Many astronomers felt the phenomenon ought to be predictable, but the existing models were not succeeding. Past storms in 1833, 1866 and 1966 (and earlier in history) showed up at times and/or places when none was expected, and failed to show up when expected in 1899 and 1932-33.
Leonid meteors are known to have originated from Comet Temple-Tuttle because they share an orbit very similar to that of the comet, with a period of about 33.2 years. In models used by most astronomers, meteors are ejected from the nucleus of the comet by eruptions of jets or geysers, usually when the comet is near the Sun. However, in general, these presumed eruptions eject the meteors at unknown times, in unknown directions, and with a broad range of velocities. So their subsequent evolution is difficult to predict. Most predictions were based on the assumption that the meteors remain in or near the orbital plane of the comet, and clustered preferentially in certain quadrants relative to the comet. But these predictions, based on when past storms appeared, were invariably unsuccessful. If the predicted times (e.g., when the Earth crosses the plane of the meteors) were off by, say, 12 hours, then the opposite side of the Earth would be turned toward the storm when it happened.
Lyytinen’s approach differed fundamentally from all others in that he applied the “satellite model” for the nature of comets, a corollary of the exploded planet hypothesis. In that model, asteroids of all sizes between dust grains and the comet primary nucleus are in stable orbits around that nucleus, inside the comet’s gravitational sphere of influence. Each time the comet approaches the Sun, the radius of the comet’s sphere of influence shrinks due to competition with the Sun for gravitational control. Many meteors find themselves outside the comet’s sphere of influence and escape the comet nucleus into solar orbits of their own. Because these escape speeds are very low, the resulting orbits are very close to the comet’s own orbit; and the escaped meteors have very little tendency to disperse.
These features of the “satellite model” for comets readily explain why the escaped meteors have such a wide range of sizes – up to a few meters in diameter, with masses of many tons – even though gentle cometary jets or geysers would have a difficult time ejecting objects larger than grain or pebble size. It also explains why the meteors stay clustered over long periods. Both are properties of a debris cloud orbiting a nucleus. More importantly, this orbiting debris cloud model, itself originating from the explosion of a planet or Moon-sized parent-body, requires certain very specific escape conditions. Meteors can only escape from their orbits around the nucleus through the so-called “Lagrange points” named “L1” and “L2”, which might be thought of as balance points between the Sun’s gravity and that of the comet nucleus. L1 is located between the comet and the Sun, and L2 is located on the opposite side of the comet. Escapes from the comet nucleus then occur through the L1 or L2 points when the comet is close to the Sun, with meteors moving in specific directions, with specific speeds, under conditions entirely determined by the gravitational forces acting. This makes tracking meteor streams following escape just a matter of computer calculations. And if the model is correct, both the times of the encounters with Earth and the densities of these escaped, invisible streams of meteors would be accurately predictable. Esko Lyytinen described this model in detail in his article.
The prediction for November was completed only a short time before publication of our September issue, and was rushed into print because that was the last chance to get the prediction documented before the event took place. Revisions of the article were occurring right up to the day the issue was sent to the printer; and in fact, Lyytinen’s last batch of minor editing corrections arrived too late to be included. But his prediction was specific and final for the fact that a storm would occur on November 18, and that the peak time would be 2h10m±10m UT. This made the optimum location for viewing the storm near 35° east longitude. Based on that expectation combined with weather and political considerations, Eclipse Edge planned and launched an expedition to the island of Cyprus in the Mediterranean.
Lyytinen’s analysis continued after the article’s publication, and in late October, he sent his final prediction for the specifics of the two meteor streams expected to contribute to the November 18 event. See Fig. 1, which shows the separate contributions for the meteor stream that escaped the comet in 1899, three revolutions ago, and for the meteor stream that escaped the comet in 1933, two revolutions ago. The latter was a minor contributor, expected to peak at about 1h40m±15m UT.
The vertical axis shows meteors per hour under ideal viewing conditions (clear skies, no Moon, radiant in the zenith), called the “zenith hourly rate” (ZHR). A similar plot was posted at <http://www.sci.fi/ ~fmbb/astro/meteorit.htm> to document the prediction.
Earlier, in an October 9 e-mail message to astronomer David Asher, another predictor of the Leonids, Lyytinen wrote: “For 1999, this model predicts in the ‘simple’ form ZHR’s of 5500/3rev. ; >500/2rev.; 160/4rev trails.” I posted the first of these predictions to USENET newsgroups sci.astro and sci.astro.amateur on October 20, with the following statement: “The Leonid meteors are predicted to reach storm levels in the pre-dawn hours of November 18 over the Middle East. We have selected a site in the Cyprus mountains for optimum viewing of the predicted peak rate of 5500 meteors per hour. (That is not a typo!) This should be more spectacular than any predictable astronomical event short of a total solar eclipse.” So it is clear that Lyytinen’s most specific prediction was for a storm with intensity in the range of 5500-7000 meteors per hour, with an uncertainty of up to ±3000/hr.
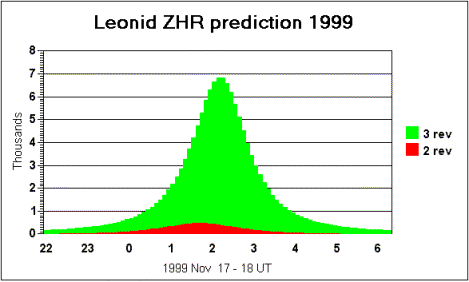
Fig. 1. Lyytinen’s predicted zenith hourly rate for the November 18 event, resulting from the 2-comet-revolution stream (escaped in 1933) and the 3-comet revolution stream (escaped in 1899).
By contrast, mainstream predictions were widely scattered. NASA sent out seven groups to make observations worldwide to ensure that observations would be made almost no matter where the peak meteor rates might happen. Most of the predictions by mainstream astronomers clustered around 3-4h UT, when the Earth passed through the comet’s orbital plane. However, the team of Asher & McNaught, cited by Esko Lyytinen in his MRB article, used a method similar to Lyytinen based on individual meteor streams released one per revolution, estimating ejection velocities by fitting to past Leonid storm data. As a result, their predicted time, 2h 08m UT, was similar to Lyytinen’s. However, their predicted rate was far more uncertain, and several possible rates ranging up to 2000 meteors per hour were suggested. In their last article in early November, Asher and McNaught predicted a ZHR of 500 meteors per hour.
Despite the fact that Eclipse Edge announced its expedition to Cyprus to observe the predicted storm only 10 weeks in advance, 18 people signed up, joining some agency people and members of the local media at our observing site in the Cyprus mountains. Because we were surrounded by mountains (see site photo in Fig. 2), no artificial illumination affected sky conditions at this site in any direction. We came prepared for meteor observing, complete with pool mattresses to stretch out on for a maximal sky view, pillows, blankets, snacks, red-cellophane-covered flashlights, plus cameras and recording equipment of all sorts.
The agenda for our four days in Cyprus included a tour of historic and archaeological sites and a four-part astronomy program, with lectures on the exploded planet hypothesis, the Leonid meteors, the latest on Cydonia at Mars, and a collage of new astronomy from cosmology and the speed of gravity to future expeditions. But without doubt the possible meteor storm was the most anticipated part of the program. The radiant rose above the horizon a bit after midnight,
with the Moon setting two hours later. The evening skies were quite clear, and the Leonids lecture covered all aspects of their history, predictions, and viewing considerations. Participants knew that no Leonid meteor storm had ever before been successfully predicted. They also knew of the remarkable string of prediction successes made by the exploded planet hypothesis, including satellites of asteroids, satellites of comets, and salt water in meteorites, to mention a few of the most recent ones. The eph would be tested again that night because it alone,
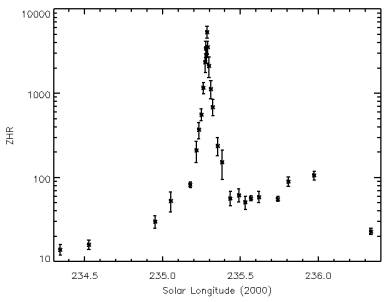
Fig. 3. IMO plot of observed meteor rates (ZHR) on a logarithmic scale. The peak shown is about 5500 meteors per hour at 2h 05m UT.
among all the prediction models, could be confident that its predicted time and rate of meteor maximum would be on target.
By local time in Cyprus, the storm maximum was expected at 4:10 a.m. We arrived at our observing site at about 2 a.m., set up, and commenced observations. The skies were cloud-free and magnificent. Frequent meteors were already in evidence. At around 3 a.m., the estimated rate was in excess of 500 meteors per hour, making it by far the most intense meteor shower ever seen by any of the participants. As the radiant climbed in the sky and the predicted storm maximum grew closer, the meteor rate was clearly increasing rapidly. The occasional fireball punctuated the activity. A few of those left visible trains that hung in the sky for up to ten seconds.
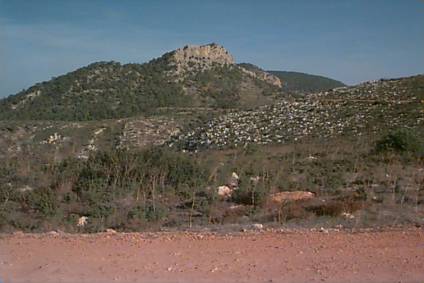
Fig. 2. Observing site for Eclipse-Edge-sponsored Leonids meteor storm expedition to Cyprus.
At about 3:20 a.m., a bank of clouds rose over the mountains to our west and began drifting our way. Within ten minutes, the skies had become almost completely overcast. We packed up our belongings and reboarded our bus, then began an adventurous ride through the dark mountains along dirt trails, trying to travel east faster than the clouds to regain clear skies. The mountain trails offered few options for travel direction, and we were forced to go well to the south before we could turn east. About every ten minutes, I hopped off the bus to check the skies. Although there would typically be just one or two small breaks in the clouds, I could see meteors in those breaks, indicating that fireworks were indeed going on in the skies.
Finally, at a quarter past 4 a.m., we realized we would not be able to outrun the clouds before the storm was well past maximum. So we headed for the nearest large clearing visible from that spot. At 4:20 a.m., we had perhaps 7-8 minutes of visibility through a drifting opening in the clouds high in the sky that allowed limited viewing of the spectacle taking place. By my estimate, the opening covered 10-15% of the sky. At 4:20 a.m., we could see an average of 8 meteors per minute through it. This translates into a ZHR of about 4000/hour. The rate was clearly declining during the minutes we watched. We had missed the storm maximum, but saw enough to know that we had come to the right part of the world and had confirmed (in broad terms) the eph-based prediction.
Within a few days, reports had come in from all over the world, and the International Meteor Organization (IMO) had posted summaries at <http://www.imo.net/leo99/leo99index.html>. Fig. 3 shows a summary plot of meteor rates (ZHR) versus solar longitude (equivalent to time). The observed peak is at about 5500 meteors per hour at a time of 2h04m±5m UT. A summary comparing Lyytinen’s prediction with observations appears in Table I.
The prediction was not perfect because the actual storm was of shorter duration than in the Lyytinen prediction. Duration was a parameter that Lyytinen had estimated by fitting to past storms. That defect can be corrected for future work.
However, it seems clear that the underlying model works very well indeed. Individual meteor streams are released at low relative speeds each time a comet approaches the Sun. If the Earth runs through one of these meteor streams, a storm results. Knowing this, we can solve other mysteries. Comet outbursts (sudden flare-ups by many orders of magnitude, often accompanied by a swelling of the coma) are such a mystery. Collisions with small asteroids would produce such an effect; but outbursts are more common than the number of small asteroids thought to be available. Now we see that comets can run into their own meteor streams with enough frequency to produce just such outbursts.
In summary, Esko Lyytinen’s prediction of the 1999 Leonid meteor storm was by far the most accurate made by any astronomer. It was quantitatively correct to within the stated uncertainties for peak time, hourly rate, and 2nd peak due to a second meteor stream; and qualitatively correct for the relative number of bright versus faint meteors. NASA announced that Asher and McNaught had the best prediction on the basis that their predicted time, 2h08m UT, was closest. However, this is not significantly different from the Lyytinen predicted time. And Asher and McNaught’s rate prediction (500/hr in their last estimate) was too low, falling more than an order of magnitude short of the observed rate.
Our congratulations to Esko Lyytinen for this history-making success!
Lyytinen Prediction | Observed | |
Peak time | 02h10m±10m | 02h04m±5m |
Peak rate | 6800±3000/hr | 5500/hr |
Content | Few fireballs | Few fireballs |
2nd peak time | 01h40m±15m | 01h53m±5m |
Table 1. Comparison of prediction and observations. |